Revolutionizing disease treatment with biotechnology and functional molecules
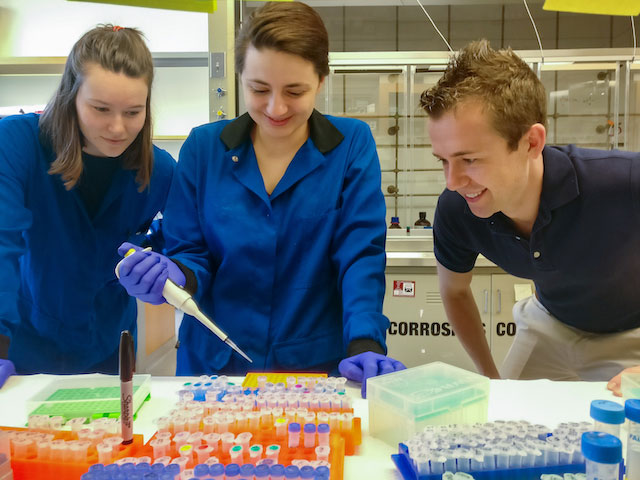
A collaborative atmosphere among world-class researchers is one of University of Chicago’s biggest strengths. Such an environment has allowed Bryan Dickinson, PhD, an associate professor of chemistry, to flourish in biological research and tackle looming challenges such as developing treatments for various diseases using the tools of his trade.
But what is a chemist doing in the world of biology?
The Dickinson lab specializes in creating functional molecules big and small to solve biological problems. Their molecular repertoire ranges from small probes to observe how fat molecules modify proteins, to virus-manufactured biosensors that peer into the inner workings of a living cell. It is precisely Dickinson’s background in synthetic chemistry and protein engineering that gives his group an advantage—they have the ability to design and produce specialized molecules as needed, depending on the research problem at hand. Dickinson brings to the table expertise in molecular technology development to enrich the vibrant medical research at UChicago.
RNA: Ready, “n”, action!
RNA molecules are bite-sized, portable copies of activated genes from the DNA. A common description of the RNA from a typical biology textbook is that RNA is the messenger between the DNA and the protein production line. RNA molecules feature heavily in Dickinson’s research.
With world-renowned RNA sequencing expert James LaBelle, MD, PhD, to study the interactions between cancer cells and pinpoint a drug target for cancer. The group is also collaborating with gastroenterologist David T. Rubin, MD, on potential treatments for digestive diseases.
Dickinson’s latest research on RNA genetic engineering using CIRTS, published in the June issue of Cell, is currently seeking new health challenges to take on. Dickinson and Chuan He have had a field day coming up with possibilities for therapeutics development as each team deepens their understanding of RNA regulation.
Collaborating with all these different experts at UChicago has allowed Dickinson’s research to extend its reach to multiple biomedical problems wherever his technology is applicable. He acknowledges that having such a diverse cadre of researchers at UChicago has also significantly benefitted his lab, propelling his work to the heights it has attained today.
“Having the medical school with the chemistry department all on one campus is pretty unique,” Dickinson said. “We are able to obtain clinical samples, perform mouse work, and leverage the hospital’s collective expertise to push the biology as far as we want. As our specialty is technology development, we often look for collaborators to extend our technology to real-world medical applications. But in other cases, we’ve also had clinicians come to us with a particular clinical problem. If it fits in with our turf of technology development, we work together to solve that problem. It goes both ways.”