Timing is everything: Circadian clocks set the rhythm for vital functions in bacteria
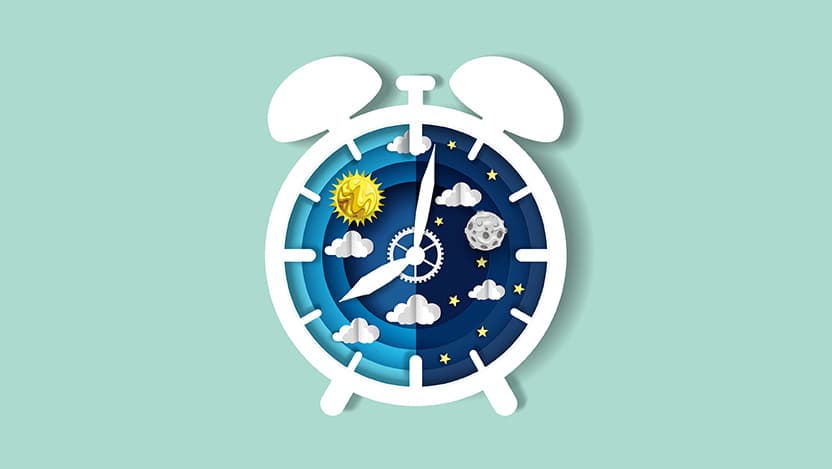
In 1938, University of Chicago sleep researchers Nathaniel Kleitman, PhD, and Bruce Richardson, experimenting on themselves, became the first to demonstrate that humans have an internal circadian rhythm.
For six weeks, they lived in Kentucky’s Mammoth Cave in complete darkness at all times. Despite having no external cues like sunlight or temperature to tell them what time it was, their daily activity patterns did not descend into chaos. Instead, the researchers both slept for about the same amount of time and woke around the same time each day, much like they would have outside the cave. They demonstrated that human activity patterns do not fully depend on the rising and falling of the sun, but that some internal process governs when we sleep and how long we stay awake.
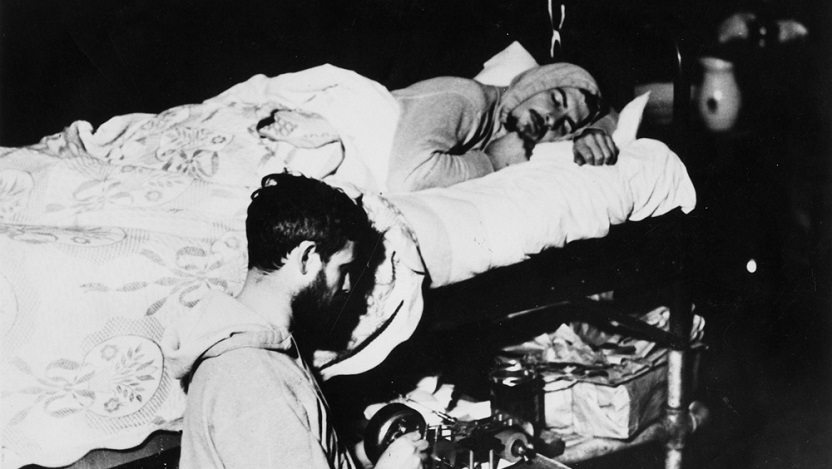
Today, scientists understand that our biology generates circadian rhythms that direct our activity and sleep to match the light and dark hours in a day, even when those external light-dark cues are absent. We also know these rhythms are very important for human health. Shift workers, who have erratic schedules that do not allow them to sleep and wake at consistent times, have a higher risk of developing cardiovascular disease, hypertension and certain types of cancer.
Circadian rhythms have been identified in countless species of every category of life, from humans to birds, butterflies to flowering plants, and even tiny single-celled microorganisms. By coordinating their daily activity patterns with the cycle of day and night, circadian rhythms help all of these species survive more successfully.
I work as a graduate student researcher at the University of Chicago in the lab of Michael Rust, PhD, Associate Professor of Molecular Genetics and Cell Biology. I study the mechanisms behind the circadian rhythms of cyanobacteria, a type of photosynthetic bacteria that live in oceans and lakes. Like plants, cyanobacteria use photosynthesis to get energy from the sun and thus benefit from having circadian rhythms to keep track of which part of a 24-hour day they can expect easy access to life-sustaining sunlight — and when they will have to manage without that energy source in the dark.
In order to be effective, the circadian rhythms of cyanobacteria need to be connected to the various physiological processes going on within themselves, like metabolism and cell division. But our understanding of what circadian rhythms regulate and of how they do so is incomplete. My graduate work aims to understand how circadian rhythms regulate changes in protein solubility according to the time of day.
One might wonder why we study circadian rhythms in cyanobacteria instead of humans or other animals. The reason is that circadian rhythms in cyanobacteria are generated by a relatively simple collection of components that can be studied and probed in ways that would not be possible in studies of other organisms. You can imagine the cyanobacterial “circadian clock” to be analogous to an analog wristwatch that can be taken off and taken apart. On the other hand, the circadian clocks of other organisms, like humans or mice or flies, are more like the clock on your phone or laptop, which are deeply integrated into their systems, and would be difficult to remove without fundamentally changing the clock or its surrounding system.
One of my research goals is to understand how and why certain proteins shift from soluble to insoluble and back, and exactly how the process is regulated by the circadian clock. Previous work done in our lab has shown that circadian rhythms in cyanobacteria influence protein solubility, causing some proteins to be more soluble during day hours and less soluble during night hours. When proteins are soluble, they are dissolved in the liquid interior of the cell, but when they are insoluble, they tend to be condensed together, with other proteins, and/or with other contents of the cell.
Recently my work has focused on a protein called KidA (yes, the Radiohead album may have inspired this name), which interacts with the proteins that generate circadian rhythms, also known as KaiA, KaiB, and KaiC. The results of a previous study from our lab showed that KidA changes its solubility from day to night, and I want to figure out how and why that happens. Does KidA need to bind to the circadian clock proteins in order to change its solubility? If we prevent KidA from binding to the clock proteins, will that change or eliminate the rhythm in KidA solubility? And if we grow cells only in the light, instead of alternating light and dark, will KidA still become insoluble at the same times?
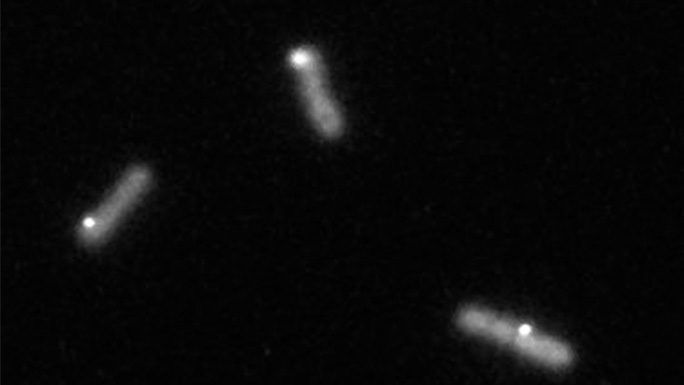
To begin answering these questions, I use time-lapse fluorescence microscopy to image cyanobacteria that produce many copies of the KidA protein with a fluorescent tag attached. We can see when KidA is less soluble because it will aggregate together in small spots that we call puncta. Those puncta assemble and disperse over the course of a light-dark cycle.
My next steps are to see what if KidA still forms puncta when I keep the lights on around the clock, or if I mutate the genes of the cyanobacteria to erase their circadian clock. In each of these cases, we might see KidA form puncta with different timing over the course of 24 hours, or KidA may even stop forming puncta altogether. The results of these experiments will tell us more about how the circadian clock regulates KidA solubility.
Further down the line, I not only want to know how certain proteins like KidA become less soluble at night, I also want to know what purpose that change in solubility serves in the cell. Maybe those proteins are becoming insoluble to be “pushed aside” when they are not needed during the night. Or maybe some proteins encounter important binding partners when they both become insoluble. Answering these questions will tell us about the specific ways in which the circadian clock influences physiological processes in cyanobacteria to help them function and survive.
When Kleitman and Richardson discovered that an internal process sets the rhythm of our activity and sleep patterns, they did not yet know where that internal process came from, or how it connected to biology or behavior. Decades later, we know so much more about how circadian rhythms are generated, and the functions they influence, but there is still so much more to discover. I hope my research deepens our understanding of how circadian rhythms allow organisms to survive and live more successfully.
Lily Burton is a myCHOICE intern, University of Chicago PhD candidate and graduate student researcher in the lab of Michael Rust, PhD, Associate Professor of Molecular Genetics and Cell Biology.

Michael Rust, PhD
Michael Rust, PhD, is an associate professor in the Department of Molecular Genetics and Cell Biology and the Department of Physics. His research focuses on understanding how the behavior of living cells is produced by the biochemical interactions of many non-living molecules.
Learn more about Dr. Rust